Beyond Traditional Insulation: How Phase-Change Materials Are Redefining Temperature Control in Buildings
Phase-Change Materials (PCMs) are specially engineered materials that absorb, store, and release thermal energy through phase transitions, typically between solid and liquid states. By utilizing latent heat, they provide efficient temperature regulation, reducing fluctuations and enhancing thermal stability. Available in organic, inorganic, and bio-based compositions, PCMs are designed with tailored melting points, thermal conductivity enhancements, and durability improvements to optimize energy efficiency.
Working Principle of Phase-Change Materials (PCMs)
Phase-Change Materials function by undergoing a transformation between solid and liquid states, absorbing or releasing large amounts of latent heat in the process. This phase transition occurs at a relatively constant temperature, making PCMs highly effective in thermal management.
When the ambient temperature rises above the melting point of the PCM, it absorbs heat and transitions from a solid to a liquid state, effectively storing thermal energy. As the temperature drops below its freezing point, the PCM solidifies, releasing the stored heat back into the environment. This cyclical process helps in maintaining stable indoor temperatures, reducing dependency on artificial heating and cooling systems, and enhancing energy efficiency in buildings.
Types of Phase-Change Materials (PCMs)
PCMs are classified based on their chemical composition into three primary types:
Organic PCMs:
- Paraffins: Derived from petroleum, paraffins are widely used in construction due to their chemical stability, non-corrosive nature, and long-term reliability. They have a relatively low thermal conductivity, which can be improved through additives or encapsulation. They are hydrophobic, preventing moisture absorption, but require proper encapsulation to avoid leakage.
- Fatty Acids: These bio-based PCMs provide excellent thermal performance and high latent heat storage capacity. They are biodegradable and have a stable phase change over repeated cycles. However, they are more expensive than paraffins and may emit odors during phase transition. Their ability to self-nucleate prevents supercooling, making them highly effective in thermal applications.
- Sugar Alcohols: Less commonly used but highly efficient, these materials provide high latent heat storage and stability over multiple cycles. However, they are prone to supercooling and phase separation, requiring advanced stabilization techniques.
Inorganic PCMs:
- Salt Hydrates: Among the most commonly used PCMs in buildings, salt hydrates offer high energy storage density and affordability. However, they tend to suffer from phase separation and supercooling, requiring stabilizers or thickening agents to maintain efficiency. They have better thermal conductivity than organic PCMs, making them suitable for applications where rapid heat transfer is needed.
- Metallic PCMs: These advanced PCMs have excellent thermal conductivity and durability, making them ideal for high-temperature applications. They are more stable than salt hydrates but are expensive and heavy, limiting their widespread use in construction. Examples include gallium-based alloys and low-melting-point metals.
- Eutectic PCMs:
- These are specifically formulated blends of organic-organic, inorganic-inorganic, or organic-inorganic materials that create a compound with a well-defined melting point. They offer a balance between the advantages of both organic and inorganic PCMs while minimizing their drawbacks.
- Eutectic PCMs exhibit consistent phase change behavior, reduced supercooling, and improved stability over repeated cycles, making them suitable for applications requiring precise thermal control.
- Common eutectic PCM combinations include paraffin-fatty acid blends and salt hydrate-organic compound mixtures.
PCMs can also be categorized based on their mode of application:
- Passive PCM Systems: These systems operate without any external energy input. PCMs absorb and release heat naturally, responding to temperature fluctuations in the environment. Examples include PCM-infused walls, ceilings, and flooring that passively regulate indoor temperatures by absorbing excess heat during the day and releasing it at night. These systems are particularly beneficial in reducing reliance on artificial heating and cooling, thereby improving energy efficiency in buildings.
- Active PCM Systems: These systems incorporate external mechanisms, such as fans, heat exchangers, or pumps, to control the thermal behavior of PCMs. Unlike passive systems, active PCM systems allow for better regulation of heat absorption and release, making them more suitable for applications requiring precise temperature control. Examples include HVAC-integrated PCM storage units and thermal energy storage systems, which optimize heating and cooling efficiency in commercial buildings.
Applications of PCMs in Building Construction
PCMs are used in various construction applications to enhance energy efficiency and indoor comfort:
- PCM-Enhanced Walls: Integrated into wall panels to moderate indoor temperature fluctuations, reducing reliance on heating and cooling systems.
- PCM in Floors and Ceilings: Incorporated into flooring materials and ceiling panels to store heat during the day and release it at night, beneficial in extreme climates.
- PCM in Concrete and Mortar: Microencapsulated PCMs mixed into concrete enhance thermal mass, reducing temperature variations inside buildings.
- PCM-Infused Gypsum Boards: Used in interior partitions to improve thermal stability without the need for extra insulation.
- PCM in Building Envelopes and Roof Insulation: Acts as a heat barrier, preventing excessive heat gain in summer and heat loss in winter.
Advantages of Using PCMs in Building Construction
- Helps in maintaining stable indoor temperatures, reducing extreme fluctuations.
- By decreasing the reliance on artificial heating and cooling systems, lower energy consumption.
- Reduced energy use translates into lower greenhouse gas emissions.
- By minimizing thermal stress, PCMs help extend the lifespan of building materials.
- Offer superior thermal regulation without requiring bulky insulation layers.
- Can be incorporated into various construction materials, including concrete, plaster, and insulation panels.
- Enhances occupant comfort by preventing sudden temperature changes.
- Reduces peak energy demand, easing the load on HVAC systems.
- Improves overall building energy efficiency, lowering operational costs.
- Provides long-term performance with minimal maintenance requirements.
- Can be tailored to specific temperature ranges for different climate zones.
- Enables passive heating and cooling, reducing reliance on mechanical systems.
- Helps prevent overheating in buildings with extensive glazing or exposed roofs.
- Enhances fire safety when combined with fire-resistant encapsulation materials.
- Can be integrated into prefabricated building elements for faster construction.
Challenges and Considerations
Despite their advantages, PCMs come with certain challenges that need to be addressed for widespread adoption. One of the primary concerns is high initial costs, as PCM-infused building materials are more expensive than conventional alternatives. Additionally, some PCMs experience phase separation and degradation over time, reducing their long-term effectiveness. Supercooling issues are another limitation, particularly in inorganic PCMs like salt hydrates, where the material does not solidify at the expected temperature, affecting performance. To counteract these issues, PCMs require proper encapsulation techniques, such as micro or macro-encapsulation, to prevent leakage and ensure durability.
Market Potential
According to Straits Research, the global Phase Change Materials (PCM) market is set for substantial growth, projected to expand from USD 839 billion in 2024 to USD 3,193 billion by 2033 at a CAGR of 16%. This growth is driven by increasing demand for energy-efficient solutions across industries, particularly in construction, HVAC, cold chain logistics, and thermal energy storage. In the construction sector, PCMs are integrated into insulation, walls, and roofing systems to enhance thermal regulation and reduce reliance on HVAC systems.
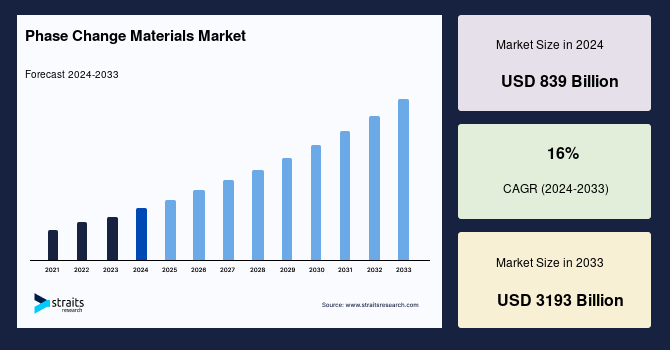
Future of PCM in Construction
As material science advances, PCM is becoming more refined, cost-effective, and efficient. Research is focusing on bio-based PCMs derived from renewable sources, offering environmentally friendly alternatives with enhanced thermal properties. Improved encapsulation methods are being developed to enhance durability and prevent material leakage. Furthermore, hybrid PCM solutions combining organic and inorganic materials are emerging to optimize thermal storage performance, addressing some of the limitations of single-component PCMs.
Government policies and industry regulations are also promoting the adoption of energy-efficient building technologies. As net-zero energy building concepts gain traction, PCMs are expected to play a pivotal role in achieving energy efficiency goals. The integration of PCM-enabled smart building systems, where sensors and automation adjust PCM activation based on real-time temperature data, represents the next frontier in intelligent thermal management.
Images- greenbuildingadvisor.com, mdpi.com,.researchgate.net